0x5.2 LOG: How to Blow Up an RBMK-1000
How Does Nuclear Energy Work and the Introduction to Novel Risks
This is part 2 of a series of articles I am writing about the Chernobyl accident and exploring if there are lessons we can take from the accident to better manage digital risks. Want to start from the beginning? Check out:
Our success as a species can be attributed to our ability to harness energy. From the first man-made fires that kept us warm to the taming of animals to outsource work, we have thrived from our ability to exploit energy from the classic elements of earth, water, wind and fire. However, it was when we figured out how to combine the energy of the classical elements, is when our capabilities went exponential.
The industrial revolution was powered by our ability to convert and transmit energy into mechanical work through boiling water to create steam. However, by the early 1800s, Michael Faraday had discovered the relationship between magnetism and electricity and theorized that electricity could be generated and used to transmit energy through electromotive forces. The first commercial electric generator was brought online in 1844, and the world’s thirst for electricity has never waned.
I am NOT a nuclear engineer. But, I may have stayed at a Holiday Inn Express…
I love learning, researching and even got to interview someone from the Idaho National Laboratories, but I am definitely not a nuclear engineer and certainly have gotten something wrong in the post. If so, leave a comment!
A Novel Energy Source
As the world began to electrify, it was mostly generated by burning hydrocarbons. While relatively cheap, the cost to extract, process, transport and burn relative to their energy density had reached their upper limits fairly early on and the need for a more efficient and cleaner energy sources were highly desired.
In the 19thth century, scientists were getting a better understanding of all the stuff around us, and more specifically the composition of that stuff. This of course was the birth of atomic theory. By the 20th century, scientists had theorized that the stuff in our world, also known as matter, is composed of infinitesimally small things called atoms. Those atoms are composed of even smaller particles known as protons, neutrons and electrons which were held together by an invisible force called The Strong Nuclear Force.
Initially applying their understandings of alchemy chemistry, they theorized that if this nuclear force could be broken, much like breaking chemical bonds, there would be a release of energy. However, it was further theorized that the release of energy had the potential to dwarf anything we had ever seen, naturally catching the eye of the military and eventually leading to the development of nuclear weapons.
During the epoch of nuclear physics and the development of the first atomic bomb, it was understood that if the fission of atoms could be both sustained as well as controlled, there was the potential for an exponentially more efficient energy source to boil water, create steam and turn turbines.
How a Nuclear Reactor Works
A nuclear reactor is where nuclear fuel, in ideal conditions, reacts with itself in a controlled manner, splitting its atoms and releasing energy. The first nuclear reactor was called “The Pile” and was stashed under the University of Chicago’s stadium bleachers. It was primitive, lacked any radiological shielding or cooling systems and outputted 0.0000005% of an RMBK-1000 power capacity. However, it proved out the theory of criticality and was the basis for all other reactor designs.
Now we are going to get a little more technical, but I promise, no math and no equations.
The Fuel
Uranium is the 48th most abundant element in earth’s crust. It isn’t much to look at, but is very special none the less, because it has the tendency to fall apart. The falling apart, also known as nuclear decay, is a natural process where unstable isotopes changing to more stable ones and sometimes even to different elements all together and in the process creates outputs energy. The ability to break the Nuclear Strong Force and fall apart is know as being fissile and is exactly what is needed to start and sustain a nuclear reaction.
All elements have variations of themselves called isotopes which reflect the number of neutrons that exist within its nuclei. The larger and more neutrons an nuclei possesses, the more unstable or fissile it will be. Uranium nuclei are quite large in mass and while most nuclei contain 142 neutrons, Uranium isotopes can contain between 122 to 150 neutrons (depending on when you look). Uranium’s most stable and common isotope is U-238, which makes up 99% of all Uranium we can mine, but while being naturally radioactive, is not fissile.
U-235 on the other hand, is the much rarer isotope of natural Uranium. It has 3 fewer neutrons and is fissile. Unfortunately, it is rare and for every kilogram of pure Uranium you have, 928 grams of it is U-238 and a mere 72 grams are U-235. Because natural Uranium is not useable in most reactor designs, it must go through an enrichment process to increase the amount of the rarer and fissile U-235 before it can be used for fuel. This is an expensive and energy intensive process, conceptually similar to distillation.
Fission the Fissile
Nuclear decay affects most elements on this earth. However, because most elements are quite stable, it is virtually unobservable to us mere mortals. Fission is a form of nuclear decay, but is almost non-existent in nature and only happens when us humans get involved.
As discussed above, a nucleus of U-235 is unstable, fissile and prone to fission if a spurious proton hits the nucleus at just the right speed. When this happens (all within about 10−20 seconds) the falling will occur:
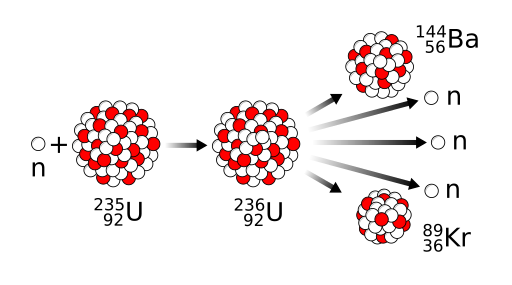
The proton is absorbed
The atomic weight of the atom increases
The atom becomes less stable and splits
The splitting tears that Strong Nuclear Force apart resulting in
Two new atoms, in this case Barium-144 and Krypton-89 and…
The release of an immense amount of energy in the form of heat and radiation
Seeking Criticality
The nuclear reactors rely on a substantial amounts of math to operate. However, the complex mathematics is not to know what is going on with any certainty… But rather to predict through probabilities what is “likely” happening within the reactor. Let that sink in.
Case in point: It was initially theorized with a bunch of math and eventually validated with “The Pile” that if you had enough of a fissile material sitting close enough together, it not only would react with itself, but it could even self-sustain the reactions, known as criticality. The calculated amount of fissile material needed to reach criticality is known as the critical mass. The amount of U-235 within the nuclear fuel is directly proportional to the mass needed to reach criticality.
The Moderators
Spurious neutrons bounce around a nuclear reactor at the speed of light. At such speeds, the neutron is more prone to bounce off of U-235 nuclei rather than be absorbed. To increase the likelihood of absorption and consequential fission, neutrons need to be slowed down or moderated.
Moderators can be made from a few different materials with the main desired property of being able to slow neutrons down, while not absorb them. The most common moderators for nuclear reactors is distilled water, heavy water (water with a heavier Hydrogen isotope called Deuterium) or graphite (special carbon), all with varying abilities to slow down neutrons.
The choice of moderator in a reactor design is based on the fuel grade and greatly effects the cost and safety capabilities of the system. Graphite was used in the original “Pile” reactor as well as in RBMK-1000 reactors. It is considered the cheapest option and works well with low-enriched fuels. Water and heavy water moderators are used as both a moderator and coolant and chosen based on the grade of fuel.
The Coolant
Splitting atoms creates heat, a lot of heat. Coolant is used within the reactor to transfer the heat energy out of the core through a closed-loop system. This functions both as a safety system to prevent melt downs, as well as to transfer that energy to heat exchanges to start the age old process of boiling water and turning turbines.
Water and heavy water are the most common coolants in nuclear reactors operating today, however molten metals, salts and even gas cooled reactor designs exists and many next generation designs leaning towards molten salts.
The Control Rods
As the amount of reactions or splitting of atoms increases in a reactor, referred to as reactivity, the amount of spurious neutrons flying around also increases, exponentially. The more neutrons flying around, the more reactivity. Left unchecked, this would result in a runaway condition, generating so much energy that the fuel and reactor components would start to melt, compromising the integrity of the reactor, its safety systems, and go brrr.
Below is a nice visual of a chain reaction:
To control reactivity within a reactor, an array of control rods are distributed throughout the reactor. Made up of neutron absorbing materials such as Boron, control rods are used as a “braking system” to granularly increase or decrease the amount of neutron absorption, affecting reactivity. Lowering control rods reduces reactivity and raising them increasing reactivity. In normal operations, control rods are use to keep the reactor running in an optimal and safe state and in emergency situations used to aggressively halt reactivity and shut down the reactor.
The Second Catalyst: Novel Risks
The concept of the "Risk Society" was introduced by sociologist Ulrich Beck, who described a modern era where novel risks have been introduced, yet not well understood. Unlike traditional risks that our brains have been wired to manage for hundreds of thousands of years, novel risks introduced by our technological and scientific advancements have brought a level of complexity and unknowns that can and have exceeded our natural risk management capabilities.
Ulrich’s book dives into examples, such as how our race to industrialize resulted in environmental degradation and climate change or more recently the scale of catastrophe from the Chernobyl accident. He argued that plant operators didn’t start their shift that night with an understanding that the nuances of the RBMK-1000’s design and an edge case “safety test” could actually be unsafe and result in a disaster. Yet, due to the complexities of nuclear energy and several known and unknown variables introduced to perform the test, the plant operators did not understand until it was too late.
As I learned about the concept of the Risk Society I couldn’t help but see the parallels in our own digital world. Intricate and esoteric risks have quietly emerged alongside our technological advances, yet thanks to our pro-innovation bias and our excitement for new things, have been swept under the rug, if understood at all. I will dive deeper into the Risk Society in a future post but here are a few exciting technologies that we as a society are failing to comprehend the impacts:
Data is the New Oil: As soon as the Internet went mainstream and users started sharing their thoughts, their pictures and everything in between, that content stopped being theirs. Whether it be the proliferation of personal data or the wholesale collection there of, the cat is out of the bag and we as a society really have no idea of the unintended consequences of the data we have lost control over. From cybercriminals to marketeers to insurance companies, this abundance and availability of personal information is and will continue to have unintended consequences.
Software All The Things: In the past two decades we have evolved from a small group of companies developing software to virtually any company or individual, being able to do so. We have become addicted to fast and cheap, but not necessarily good software and further more reliant dependent on using “code downloaded from the Internet, from someone we don’t know”. The democratization of software development has brought massive innovations and benefits. However, businesses, individuals and society as a whole are being exposed to more and more risk as our society continues to rely on software to work properly in critical sectors such as infrastructure, banking and safety.
Digitization of Heavy Industry: Integrating digital technologies into heavy industry and critical infrastructure can bring major benefits to our society. However, combining the problems with Software All The Things with already dangerous industrial processes, the full comprehension of risk is not understood until it is too late. Cybersecurity threats and technical malfunctions in these sectors can result in catastrophic outcomes, including physical harm, environmental damage, and significant economic disruption.
Artificial Intelligence (AI): (And how could I forget AI!) The AI fervor from 2023 onwards has been unprecedented and if you weren’t doing something with AI, you were falling below the digital poverty line. But for all the hype and a little bit of the awesomeness that is Large Language Models (LLMs) and Generative Pre-Trained Transformer (GPTs), they have also introduced a plethora of known and unknown concerns for businesses, individuals, artists and most scary: society.
If you made it this far, great, you’re officially a nerd 😉 and appreciate technology. Subscribing (it’s free!) and sharing would be helpful on creating more nerds. #alwaysbelearning